MY RESEARCH BLOG
under construction
History of Development of Lithium Superionic Oxides
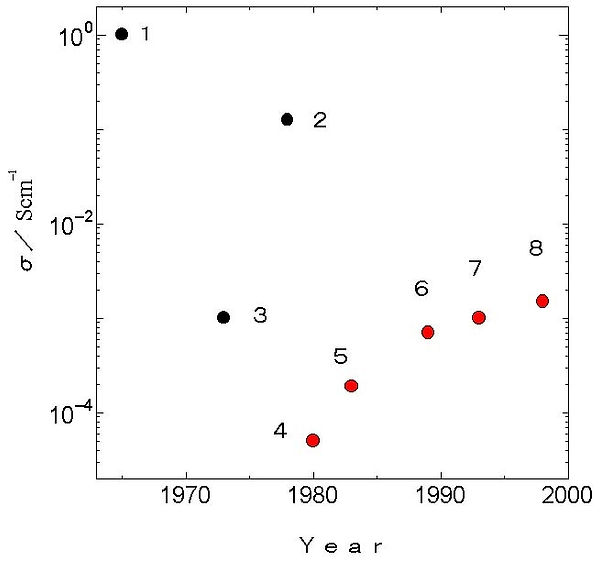
1. Li2SO4, A. Kvist and A. Lunden, Z. Naturf., 20a, 235 (1965).
2. Li4SiO4, A.R. West, J. Appl. Electrochem., 3, 327 (1973).
3. LISCON, (Li14Zn(GeO4)4), H. Y-P. Hong, Mat. Res. Bull., 13, 117 (1978).
Ambient-temperature ion conductors [Red marks]
4. Li3.6Ge0.6V0.4O4, J. Kuwano and A.R. West, Mat. Res. Bull., 15, 1661 (1980).
5. Li1+XTi2-XMX(PO4)3 H. Aono et al, J. Electrochem. Soc., 136, 590-591 (1989).
6. β-La0.5Li0.5TiO3 Y. Imaguma et al, Solid State Communications, 86, 689-693 (1993).
7. α-La0.51Li0.34TiO3 Y. Harada, Y. Hirakoso, H. Kawai and J. Kuwano,
Solid State Ionics, 121(1–4), 245-251 (1999) 245–251.
History of Development of Lithium Superionic Oxides
They can be also classified into four groups according the type of compounds:
1 and 2: Lithium oxyacid salts
3 and 4: gamma-ⅡLi3PO4 solid solutions
5: Li-substituted NASICONs
6 and 7: Li-ion conducting A-site deficient perovskites (Li-ADPESSs) Lithium-ion Conductors developed by my research group
I have developed two of the conductor group.
The first is γ-ⅡLi3PO4 solid solutions: the most famous composition is Li3.6Ge0.6V0.4O4 [4. in the figure: J. Kuwano and A.R. West, Mat. Res. Bull., 15, 1661 (1980)]. This is known to be the first ambient-temperature Li-ion conductors. We also have synthesized about twenty of the gamma-Ⅱsolid solutions based mainly on silicates, germanates and titanates with high ionic conductivity. One of their merits is that their sinterability is very good; the grain boundary resistance of the sinter is comparable to or small than the bulk resistance unlike those for Li-NASCONs and lithium-ion conducting perovskites. In addition, the silicate-based solid solutions shows good chemical stability to Li metal unlike Li-NASCONs and lithium-ion conducting perovskites. Several research groups including groups of Hitachi, NTT and US Army Research Lab applied them to the electrolyte in all-solid-state lithium solid batteries.
The second is the disordered α-La0.51Li0.34TiO3 (7. in the figure), which shows the best lithium ion conductivity (1.510-3 Scm-1 at 25℃) of known oxide-based lithium ion conductors. We have reported that the disordered polymorph undergoes an order-disorder (β-α) transition at a temperature of 1150℃. The high-temperature -form has a simple cubic perovskite cell (a=ap, space group: Pm3m) with the disordered arrangement of La3+ ions, while the low-temperature β-form is stable below 1150℃, and has a tetragonal superstructure cell (a=ap, c2ap, space group: P4/mmm), doubled along the c-axis owing to the alternate arrangement of the La3+-rich and (Li+・vacancy)-rich layers along the same axis. We have reported that the conductivity is strongly depends on the degree of the ordering: the conductivity decreases with increasing degree of the ordering, in spite of the formation of the (Li+・vacancy)-rich layers. The solid solution was first discovered in 1987 by Russian researchers; unfortunately this had attracted almost no attention until Inaguma and co-workers (6. in the figure) rediscovered the partially ordered β-form in 1993.
Applications
Batteries (Large-scaled batteries for load leveling, energy storage, EV, HEV; thin film batteries; small-scaled batteries for portable electric devices). Sensor (CO2 etc). Ion-exchangers.